Risk management and contractual risk allocation in subsea site investigations and installation activities for offshore wind farms
Friday 22 November 2024
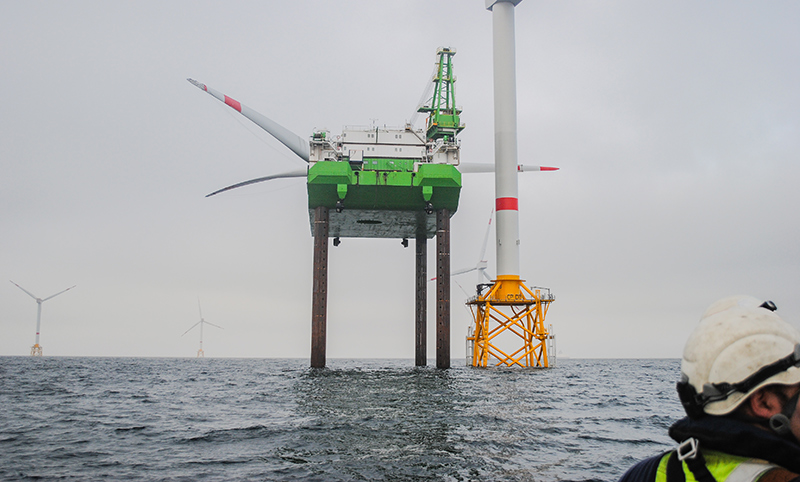
Credit: Natascha/Adobe Stock
Emilio Linde-Arias
Exponent, London
elindearias@exponent.com
Risteard de Paor
Herbert Smith Freehills Spain, Madrid
Risteard.dePaor@hsf.com
Since the establishment of the inaugural offshore wind farm at Vindeby, Denmark in 1991, there have been tremendous advancements in the field. Modern wind turbines have increased considerably in size and are now positioned further offshore. Importantly, offshore wind turbines can be much larger than their onshore counterparts and the largest have rotor diameters of nearly 300m, covering a maximum swept area equivalent to nine soccer pitches.[1] This requires cable laying, the utilisation of innovative foundation types, and poses several subsea engineering challenges with potential cost and time consequences for project developers.
This article focuses on subsea engineering-related risks and their investigation in the design stage, leaving to one side other risks such as regulatory and supply chain risks, and contracted offtake arrangements which, in sum, leave little margin for error on these projects. During the design phase, it is essential to understand the ground conditions. Site investigations thus require ground sampling and in situ tests. However, these explorations come with their own risks and challenges, potentially resulting in incomplete or inaccurate information about the ground conditions and causing delay and additional costs.
The importance of collecting geotechnical data at an early stage
Planning and implementing a geotechnical investigation for offshore wind farms introduces a unique set of complexities.
Nevertheless, some developers fail to understand the importance of collecting geotechnical data from the outset. During the planning stage of a wind project, a primary aim of subsea surveys is to secure environmental consents by identifying protected marine species and habitats. This also feeds into determining the project site location. However, these surveys typically do not prioritise the collection of geotechnical data, and the consideration of project-specific hazards, to undertake investigations and guide the design.[2]
these explorations come with their own risks and challenges, potentially resulting in incomplete or inaccurate information about the ground conditions and causing delay and additional costs
Offshore site investigation demands specialised equipment capable of operating under significant pressures and depths. Additionally, these operations are costly and time-intensive, requiring efficient strategies to minimise vessel usage and personnel deployment. These operations are planned during good weather seasons which means that specialised vessels are in high demand at the relevant times; any unexpected delays can lead to vessels moving to another project, thus compounding project delay.
Anchoring point to the seabed and adverse ground conditions
Foundations typically account for 35 per cent of the total cost of an offshore wind farm. The turbines may be supported by any of a variety of foundations (gravity base, suction caisson, monopile, tripod or jacket/lattice foundations – collectively categorised as grounded structures) or may use floating platforms through anchors that moor the turbines. Generally, grounded structures are used for shallow waters, up to 60m depth, while floating systems are used for deeper waters.[3] Monopiles, due to their simplicity in shape and straightforward manufacture, have been the industry-preferred foundation type.[4]
Geological conditions are a critical factor in choosing the appropriate foundation type. Differing or adverse ground conditions have proven to be a significant risk that can impact the installation of the turbines. For example, soils with unexpectedly low strength can necessitate deeper pile penetration for the foundation, whereas unforeseen excessively hard layers may hinder pile drivability. Even more simple gravity-based structures demand a relatively level seabed and can require extensive seabed preparation, potentially escalating project costs.[5] The anchoring of floating structures can also be affected by ground risks such as early refusal due to obstructions or presence of rock.[6]
Cable laying
Cable laying is another activity prone to disruption due to ground conditions. It involves laying and burying high-voltage cables on the seabed to connect the wind turbines to each other and to the offshore substation. Depending on seabed conditions, the level of protection or relevant type of hazard, the burial depth of several wind farm cables is typically in the range of 0.5–2 m. This process requires knowledge of the seabed composition to adopt the best installation method. Indeed, it is reported that around 80 per cent of insurance claims in these projects result from issues during cable installations, which brings cable-laying contracts into focus as an area of risk mitigation.[7]
To mitigate these ground risks, the fundamental challenge, similar to onshore projects, lies in accurately predicting ground conditions despite inherent uncertainty in the behaviour of the soil in terms of spatial variability.
Non-intrusive and intrusive techniques each pose challenges
The offshore environment itself adds layers of complexity to geotechnical site investigations, especially for seabed sampling. Non-intrusive techniques such as remote sensing and geophysical surveys, typically conducted during the planning and feasibility stages, offer a broader view of the underwater geology: the data should be sufficient to demonstrate the suitability of preferred design concepts. However, soil properties and profiles obtained with non-intrusive methods are an interpretation from the data collected and cannot be directly observed. Ground truthing with intrusive investigation of the seabed is still required for the validation of the geotechnical model.
Intrusive investigations require a deployment method. For depths under 20m, a standard rig from jack-up platforms or anchored barges can be used. In deeper or severe conditions, vessels with dynamic positioning are required. These deployment systems are exposed to harsh sea conditions such as strong currents and high waves, which can degrade data quality, delay the operations and endanger operational safety. In addition, maintaining the stability of survey vessels in such conditions is challenging, resulting in errors in positioning. Vessel selection is critical, since they are required to operate in the foreseen adverse weather. Use of an unsuitable ship can result in the termination of operations due to poor sea conditions.
Geotechnical site surveys for offshore wind projects typically require penetration to depths greater than 10m, achievable with drilling of boreholes. Depending on the ground conditions, some sampling methods are more suitable than others. For example, piston samplers are impractical for sand. In cases such as boulder clays and cemented soils, rock coring techniques may be necessary. Using the wrong technique can lead to poor sample quality or low recovery.
In situ tests allow direct measurements of specific soil properties. The cone penetration test (CPT) involves pushing an instrumented cone into the seabed using a rod. This method allows for direct measurement of specific soil properties, and it can be conducted from a platform or a vessel. However, the presence of boulders can cause early refusal, impacting the test’s effectiveness.[8]
Offshore ground investigations frequently encounter delays when unsuitable equipment is used. For instance, deploying the wrong type of vessel can lead to operational inefficiencies. Employing incorrect sampling techniques can result in poor-quality samples, which may necessitate repeating the sampling process. These issues can significantly hinder the progress of the investigation and extend the overall timeline.
Use of new technologies
In response to various challenges, the offshore wind industry has proactively adopted new technologies to improve efficiency and the quality of investigations. This includes employing remotely operated vehicles (ROVs) that can be controlled from onshore, minimising the need for specialised personnel at sea. Additionally, the industry has adapted onshore technologies like sonic drilling that can allow a continuous sampling of soft soils and coring of rocks if encountered. This method is potentially suitable for all types of geological conditions. There has also been a push to integrate multiple operational techniques into a single seabed rig, thereby reducing the time and resources spent on offshore vessels.
Innovating in offshore construction carries inherent risks that can lead to increased costs and potential budget overruns and delays if the technologies fail to deliver
Innovating in offshore construction carries inherent risks that can lead to increased costs and potential budget overruns and delays if the technologies fail to deliver. The risks include technological reliability issues, where new tools may not perform as expected, due, for example, to inadequate testing or testing under unrepresentative conditions, or to particularly harsh conditions that were not factored into the technology’s design. Implementing new methods adds complexity, requiring specialised skills needed for new technologies. Finally, unforeseen safety risks and environmental impacts in sensitive marine ecosystems can also arise.
Addressing risk in the contract
To help prevent disputes, it is crucial to thoroughly assess the risks and incorporate appropriate treatment into the contractual framework, ensuring that expectations regarding quality, timing and costs are met.
Importantly, there is still no standard form contract available which specifically deals with offshore wind projects, although FIDIC has announced that it will be publishing such a contract near the end of 2025. Therefore, most contracts in this space are (often heavily) modified FIDIC or Leading Offshore Energy Industry Competitiveness (LOGIC) forms.
Importantly, there is still no standard form contract available which specifically deals with offshore wind projects
These modifications are unsurprising, given that offshore projects are typically procured in a disaggregated manner, where multiple contracts are awarded across a range of specialist contractors and equipment suppliers, with the project owner or developer managing the work distribution rather than an engineering, procurement and construction (EPC) contract serving this function.
Concerning bad weather risk for subsea activities, the FIDIC forms entitle the contractor to an extension of time for ‘adverse climatic conditions’ which are unforeseeable relative to the climatic data provided by the employer or published for the relevant location. However, typically more detailed provisions are set out, in the marine subsea survey contract, dealing with waiting on weather days and weather windows.
For example, marine survey service contracts can include a weather downtime risk share mechanism whereby the developer agrees to grant the contractor a certain number of days schedule allowance and a lump sum payment in the event of adverse weather which impedes operations (with proportionate reimbursement by the contractor should less than the allowance end up being required). Should the adverse weather exceed the schedule allowance, the developer agrees to issue a variation to the schedule, and to increase the contract price by a certain amount for each additional day of adverse weather. Where weather windows are used to delimit responsibility, it is important to clearly define the windows and the criteria for time extension relief as well as how knock-on delays are dealt with. Clauses that seek to impose liability (such as delay liquidated damages) on one contractor due to non-concurrent delay of an interface contractor may be unenforceable as a penalty depending on the jurisdiction.
As regards subsea condition risk, marine survey contracts typically contain a clause deeming the contractor to have informed itself of those conditions, at least to the extent the information has been provided by the developer. In the case of cable-laying contracts (ie, where separate to the route survey contract), they typically contain clauses which provide for an allowance should subsea conditions necessitate cable rerouting, though this will of course be limited. The contractor also typically provides an indemnity in respect of any damage the contractor causes to the subsea environment.
In offshore site investigation contracts, it is vital to ensure that the explorations adhere to specified quality standards. Performance criteria should be clearly defined for both the quality of the outcomes (such as sample recovery rates) and the capabilities of the subsea rigs and vessels (including force and torque specifications, as well as the vessel’s resilience to adverse weather conditions). In a highly innovative environment, developers should consider whether they want to be prescriptive about the type of drilling methods and vessels used, or if they would rather transfer that risk to the market and concentrate on being restrictive with the expected results.
Notes
[1] Adnan Memija, ‘Mingyang Rolls Out “World’s Largest” Offshore Wind Turbine in Capacity and Rotor Diameter’ (Offshorewind.biz, 13 December 2023), see www.offshorewind.biz/2023/12/13/mingyang-rolls-out-worlds-largest-offshore-wind-turbine-in-capacity-and-rotor-diameter, accessed 1 October 2024.
[2] A Muir Wood and P Knight, ‘Site investigation and geotechnical design strategy for offshore wind development’, in Proceedings of the 18th International Conference on Soil Mechanics and Geotechnical Engineering (International Society for Soil Mechanics and Geotechnical Engineering, 2013).
[3] S Bhattacharya, Design of Foundations for Offshore Wind Turbines (1st edn, John Wiley & Sons, 2019). See https://doi.org/10.1002/9781119128137 , accessed 1 October 2024.
[4] Offshore Wind in Europe: key trends and statistics 2020 (WindEurope, 2020), see https://proceedings.windeurope.org/biplatform/rails/active_storage/blobs/redirect/eyJfcmFpbHMiOnsibWVzc2FnZSI6IkJBaHBBcDhDIiwiZXhwIjpudWxsLCJwdXIiOiJibG9iX2lkIn19--652a2d205034a95011d035451622eca84378db37/WindEurope-Offshore-wind-in-Europe-statistics-2020.pdf, accessed 1 October 2024.
[5] Guidance Notes for the Planning and Execution of Geophysical and Geotechnical Ground Investigations for Offshore Renewable Energy Development (Society of Underwater Technology, May 2014), see https://sut.org/wp-content/uploads/2014/07/OSIG-Guidance-Notes-2014_web.pdf, accessed 1 October 2024.
[6] Cerfontaine et al, ‘Anchor geotechnics for floating offshore wind: Current technologies and future innovations’ (2023), 279, Ocean Engineering.
[7] Claire Smith ‘Renewables demand results in new guidance for offshore ground investigation’ (New Civil Engineer, 15 July 2024), see www.newcivilengineer.com/archive/renewables-demand-results-in-new-guidance-for-offshore-ground-investigation-15-07-2014/, accessed 1 October 2024.
[8] ETR Dean, Offshore Geotechnical Engineering: Principles and Practice (Thomas Telford, 2010).